Overview
Features
- Industrial Grade Sensor Mass concentration range 0-1000 μg/m^3
- Mass concentration resolution 1 μg/m^3
- Mass concentration size range PM1.0, PM2.5,PM4.0,PM10.0
- Number concentration range 0 to 3000 1/cm^3
- Number concentration size range PM0.5, PM1.0, PM2.5,PM4.0,PM10.0
- Unique long-term stability upto 8 years
- Laser-based scattering principle and advanced algorithms.
- Inbuilt auto cleaning feature (after every 255 transmissions)
- Industrial Grade Sensor with Range 0 to 100% RH / -25°C [-13°F] to 85°C [185°F])
- Resolution 0.04% RH/0.025°C
- Accuracy 2% RH/ 0.3°C
- 2 Mile Range with On-Board Antenna
- Superior LOS Range of up to 28 miles with High-Gain Antennas
- Interface to Raspberry Pi, Microsoft Azure, Arduino and More
- Example Software for Visual Studio and LabVIEW
- Wireless Mesh Networking using DigiMesh®
- 256 Sensor Node per Network
- Power efficient Built-in Sleep mode
- User Configurable Sleep duration
- Up to 500,000 Transmissions from 4 AA Batteries
- X-bee API mode use for reliable communication
- Improved reliability incorporating Retry feature in case of packet loss
- Real time battery status
Applications
- In House Temperature Humidity monitoring
- Hospital Air quality monitoring
- HVAC application
- Storage Unit Weather Monitoring System
- Warehouse Health Monitoring System
- Component of Low Power IoT System
- Home Automation
Description
Introducing NCD’s long-range wireless Particulate Matter Sensor for Air Quality Monitoring With temperature humidity sensor, boasting up to a 28 Mile range using a wireless mesh networking architecture. Incorporating the SPS30 and SHT30 temperature humidity sensor, transmits highly accurate particle size, mass concentration, temperature and humidity samples at user-defined intervals.
PM2.5 and PM10 refer to particulate matter with particle diameter up to 2.5 microns and 10 microns, respectively, and are among the most dangerous air pollutants. Due to their small size, PM2.5 particles can travel deep into the human lung and cause a variety of health issues; for instance, by triggering asthma attacks or contributing to cardiovascular disease. The SPS30 will enable the implementation of innovative air quality monitoring devices that prevent air pollution damage. This sensor is designed to measure PM1.0,PM2.5,PM4.0 and PM 10.0.
Its measurement principle is based on laser scattering technology. This technology, together with high-quality and long-lasting components, enables accurate measurements from the device’s first operation and throughout its lifetime of more than eight years.
The on-board temperature sensor is rated for -25°C to 85°C or -13°F to 185°F and the humidity sensor is rated for 0 to 100% RH. Powered by just 4 AA batteries and an operational lifetime of 300,000 wireless transmissions, a 10 years battery life can be expected depending on environmental conditions and the data transmission interval. Optionally, this sensor may be externally powered.
With an open communication protocol this IoT wireless Particulate Matter Sensor for Air Quality Monitoring product can be integrated with just about any control system or gateway. Data can be transmitted to a PC, a Raspberry Pi, to Microsoft Azure® IoT, or Arduino. Sensor parameters and wireless transmission settings can be changed on the go using the open communication protocol providing maximum configurability depending on the intended application.
The long range, price, accuracy, battery life and security features of Long Range Wireless Particulate Matter Sensor for Air Quality Monitoring makes it an affordable choice which exceeds the requirements for most of the industrial as well as consumer market applications.
To complete a network with an industrial sensor at one end, a Zigmo/Router is required at the receiving end (PC end) that receives data from sensor. A set of sensor and Zigmo is shown in following figure.
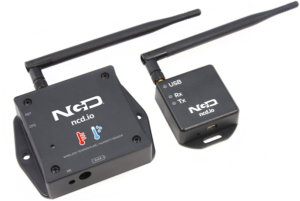
Getting Started
The sensor and Zigmo/Router come pre-programmed and work out of the box. In this section we will setup a sensor and Zigmo link and start receiving data on our PC. Though this guide shows how to visualize data on LabVIEW utility, you can also use a simple serial terminal to see raw data by following these steps.
Resources Required
- Industrial IoT Wireless Particulate Matter Sensor (with power source Battery Or External DC)
- Zigmo/Router for PC (One Router will work with Multiple Sensors)
- PC/Laptop with an OS installed or Any IoT Embedded Device
- Alpha Station PC utility
Steps
- Power-up the Wireless Sensor and make sure its antenna is installed
- Connect your Zigmo/Router to your PC
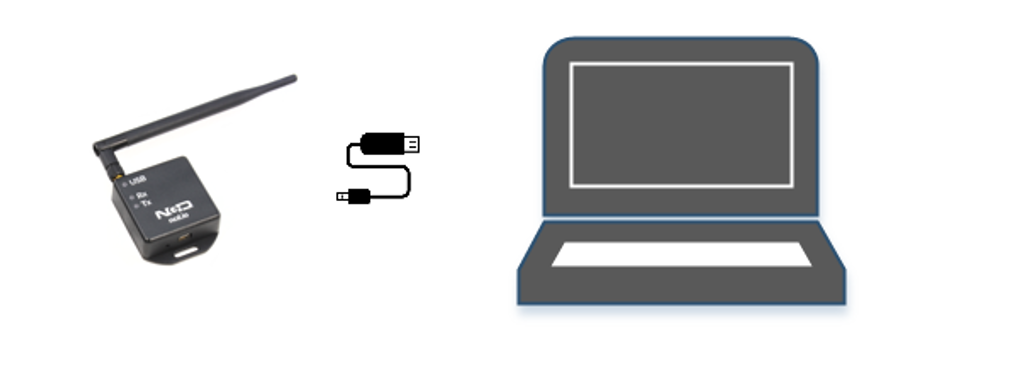
- Identify the serial port allocated to it by going into device manager (You can also find the serial port using Digi provided utility XCTU)
At this stage, both the Sensor and Zigmo have automatically established communication and the data can be read from the serial port at which Zigmo has been installed.
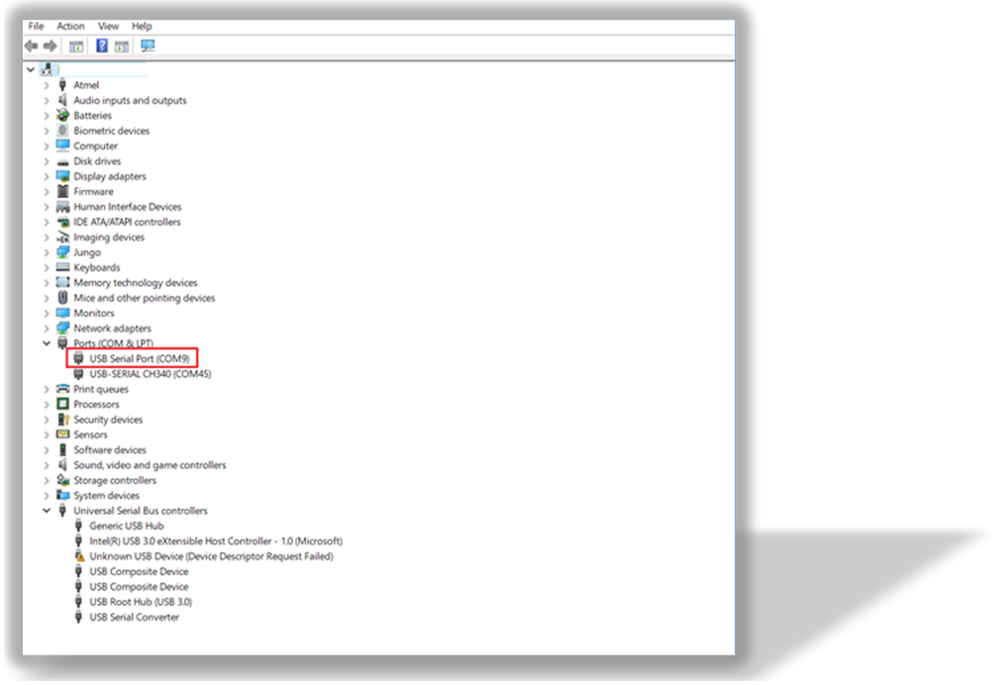
- Install the LabVIEW utility for the sensor you are working with. Run this utility.
- Press the port configure button and select the PORT you identified in step 3. Select baud rate of 115200 and press OK.
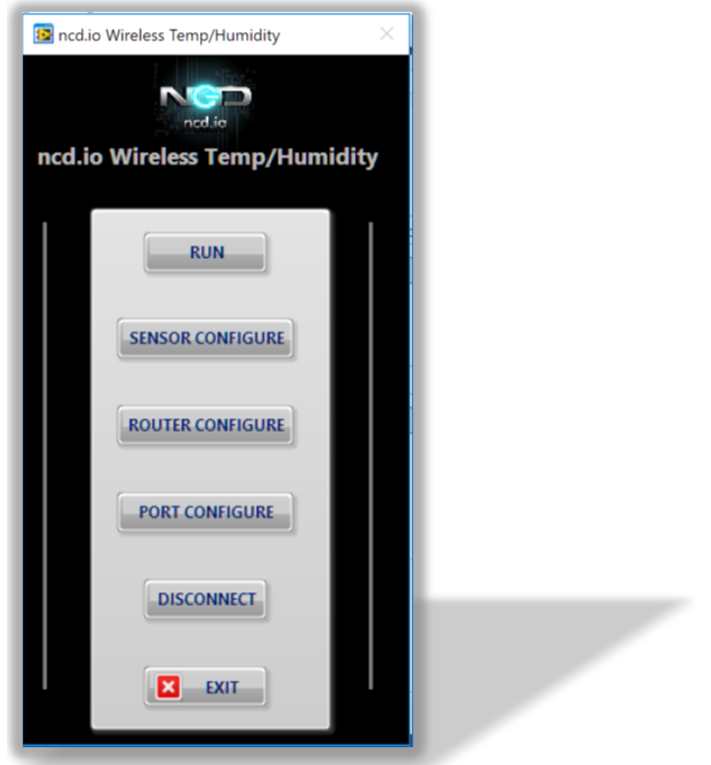
- Press the Run button to visualize incoming data
If you were not able to communicate after completing the above steps then there might be a fault at either end of the communication network. Please refer to the troubleshooting section for identifying and resolving some common issues. If you are still not able to communicate after troubleshooting then please contact us at any time.
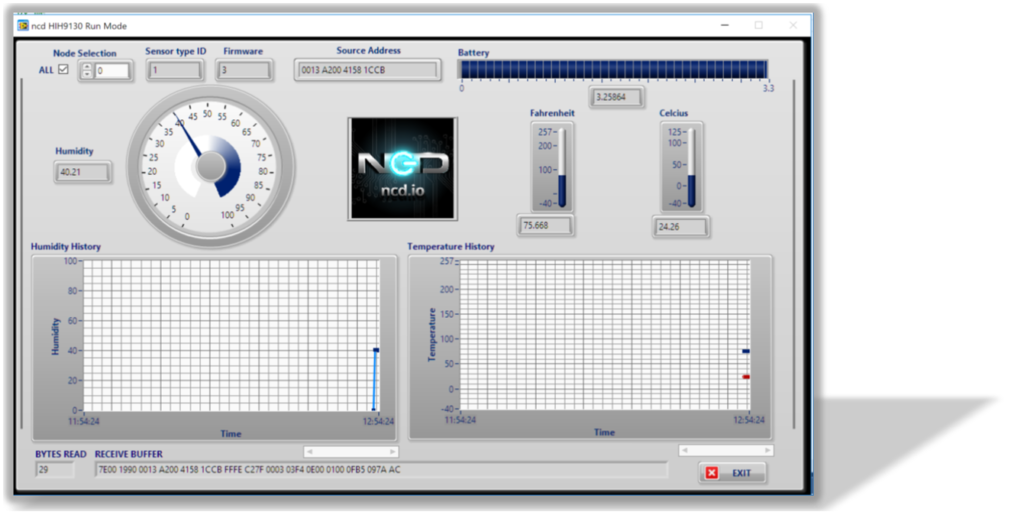
Troubleshooting
Changed/Unknown setting at sensor end
One of the issues for unsuccessful communication can be a changed setting at the sensor end due to which the sensor and Zigmo are unable to establish a connection. You can resolve this problem by going back to the factory default settings which are provided in Table 1. Please refer to Figure 7 and follow steps shown in it for applying factory default settings.
Once the sensor resets it will start sending a frame every 600 seconds after factory reset.
One of the issues for unsuccessful communication can be a changed setting at the sensor end due to which the sensor and Zigmo are unable to establish a connection. You can resolve this problem by going back to the factory default settings which are provided in Table 1. Please refer to Figure 7 and follow steps shown in it for applying factory default settings.
Once the sensor resets it will start sending a frame every 600 seconds after factory reset.
Please refer to the detailed document available to Digi website to understand X-bee communication parameters and its operation mechanism.
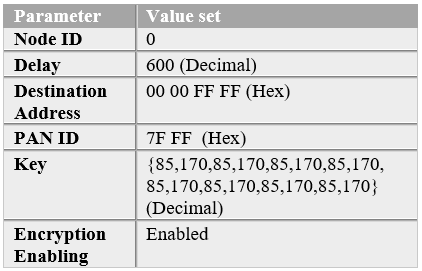
Changed/Unknown setting at PC end
Sometimes a changed setting at Zigmo end, whether intentional or unintentional, can cause a network failure and no data reception at PC end. To fix this issue when the sensor end is operating at factory default settings you will have to bring the Zigmo/Router to factory default settings as well. For that, please download the configuration file for Zigmo from our website. You will also require XCTU utility provided by Digi.
After installing XCTU Utility, run it and go to add a radio module. Select the serial port at which Zigmo is connected and press finish. This will connect the Zigmo to XCTU.
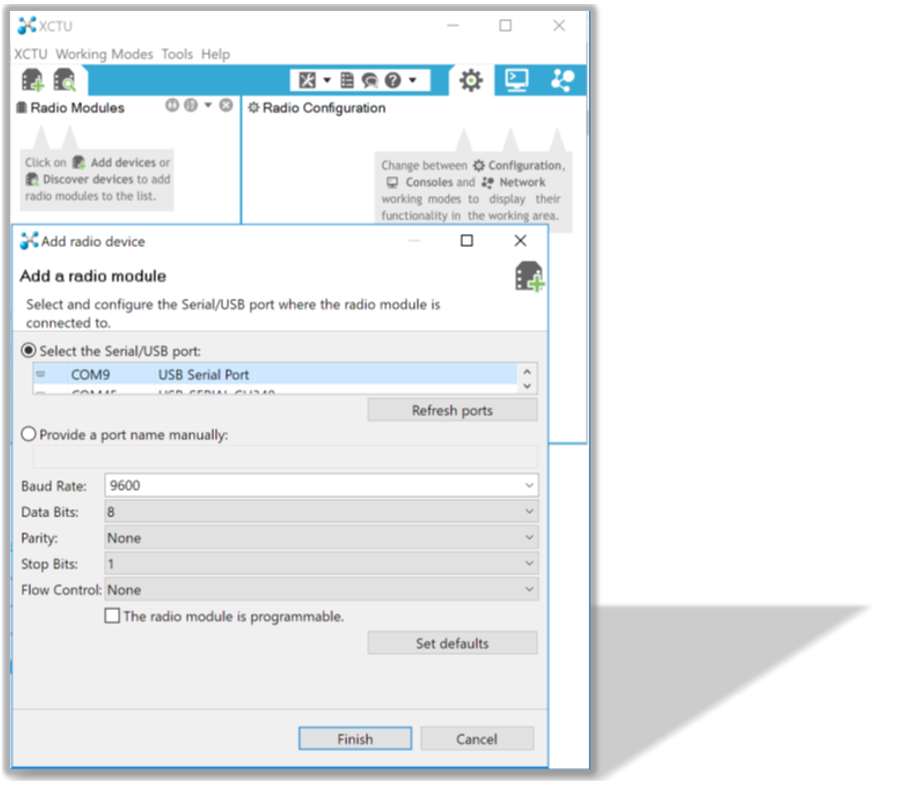
After double clicking the added module, a list of parameters will be displayed on the right side. Select the load configuration file from the top and select configuration file form the location where you downloaded it earlier.
Now press the write button on top to write these parameters. Close the XCTU utility and open the LabVIEW utility and follow the steps in getting started section to communicate with sensor.
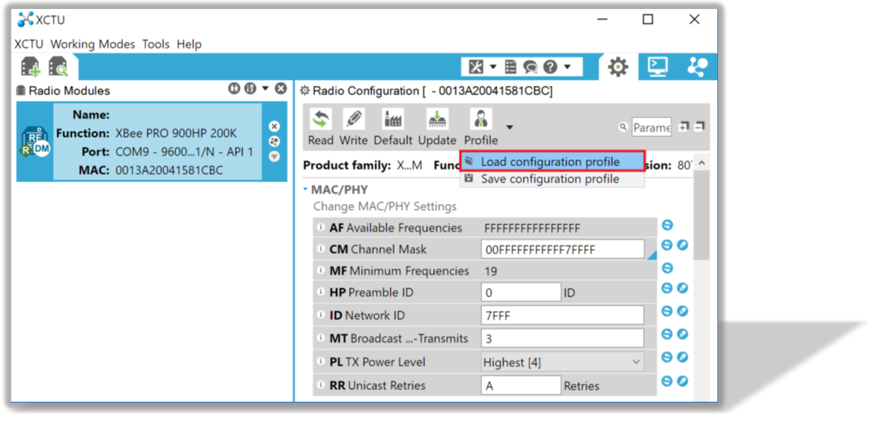
Modes of Operation
This module incorporates 2 modes of operation, these are
- Run Mode
- Configuration Mode
Run mode is the standard mode, the module will always enter Run mode if no button is pressed during Power-up/Reset. Configuration mode is intended to configure sensor parameters and the X-bee parameters on the sensor end. Note that the Sensor end X-bee is only configurable via the sensor controller using the commands provided in device manual. Figure 7 illustrates these modes.
The device sends a startup packet which can be used to determine the mode in which it is operating. These packets are shown in Table 2.
Mode Selection Process
The CFG button on the module is used to change mode. If CFG button is pressed and the module reset button is pressed, the module will enter the configuration mode. The amount of time CFG button has to be pressed is shown in Figure 7.
Note that settings only take effect after the reset.
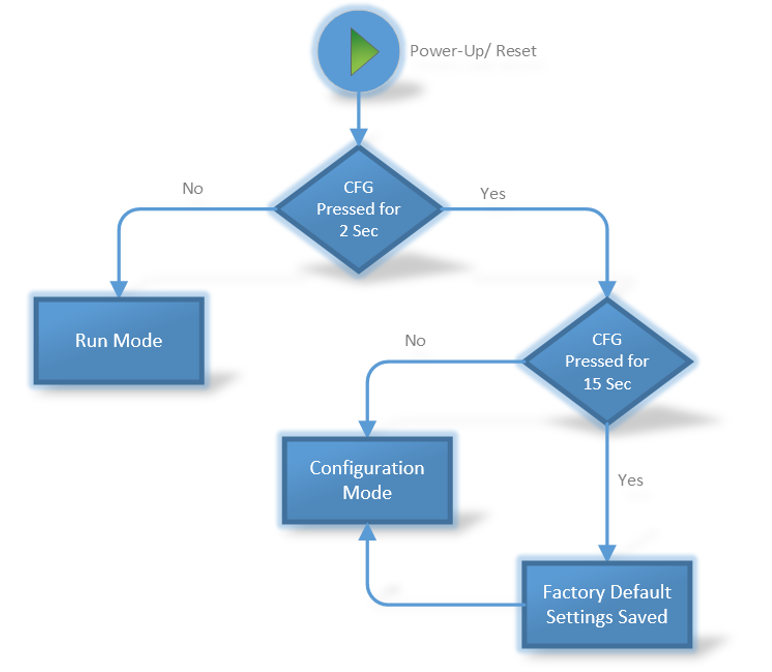
Frame Communication at Power up
In figure 8, Mode bytes highlighted in red can be compared with the values provided in Table 2 to determine the mode in which the sensor is operating. Node ID is the ID of the given sensor while sensor type determines the type of sensor. Both of these can be used to determine the exact sensor which is sending the information.
A shown in second column in Table 2, the sensor configures its PAN ID automatically depending upon the mode it is working in. During factory reset it sets the PAN ID to the value given in table therefore the factory reset frame will only be received if your Zigmo/Router PAN ID matches this ID. Please note that right after factory reset the sensor enters configuration mode therefore its PAN ID is changed again and a new frame is generated. All 3 type of frames are shown in Figure 9, Figure 10 and Figure 11.
The factory default settings are shown in Table 1. For parameter description please refer to the section on configuration.
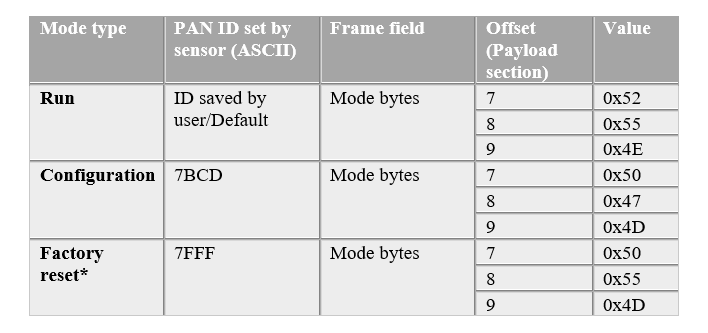
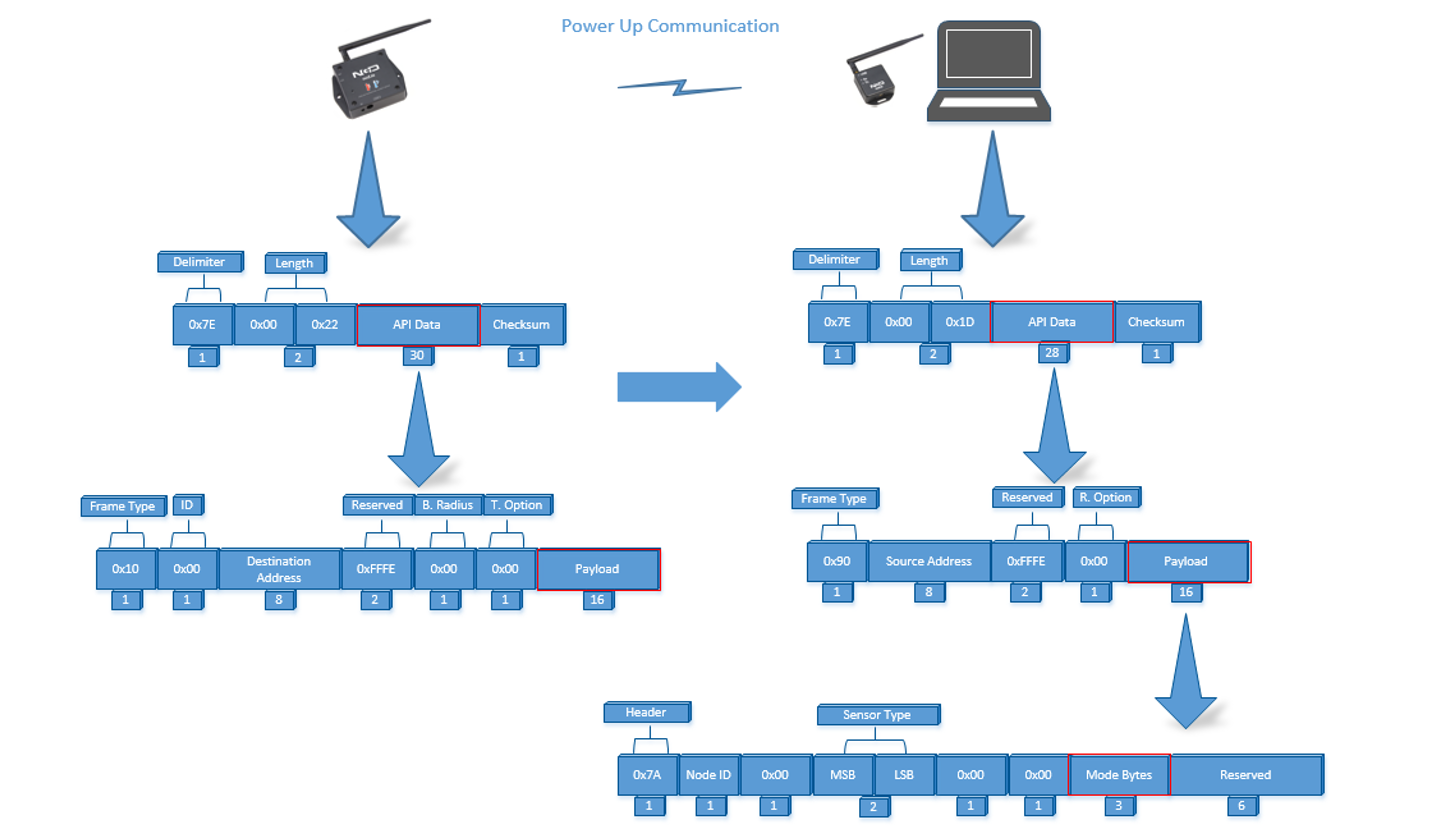
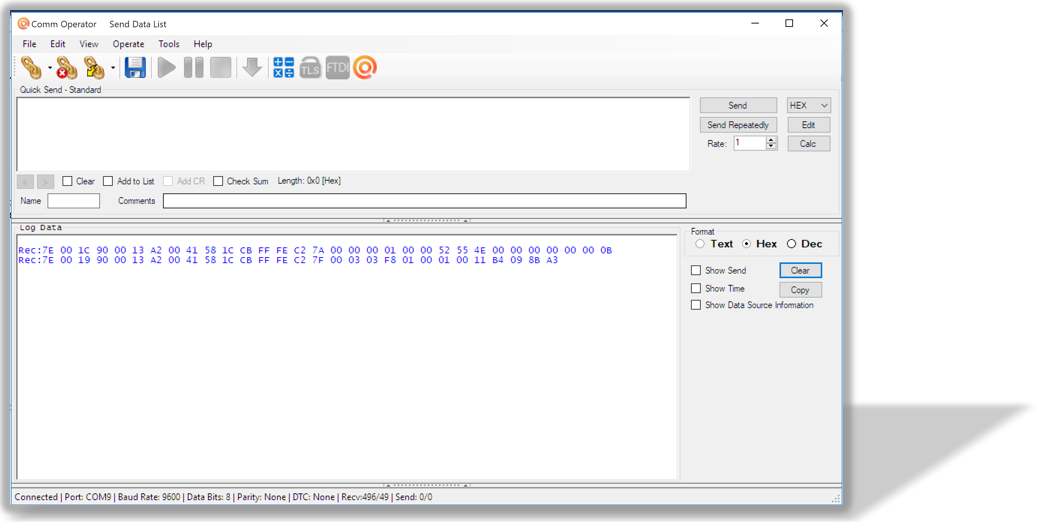
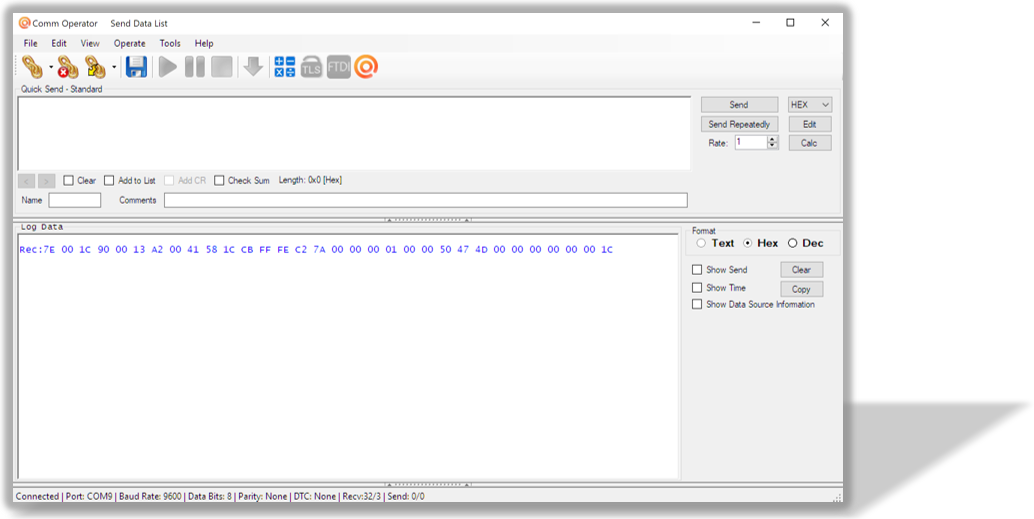
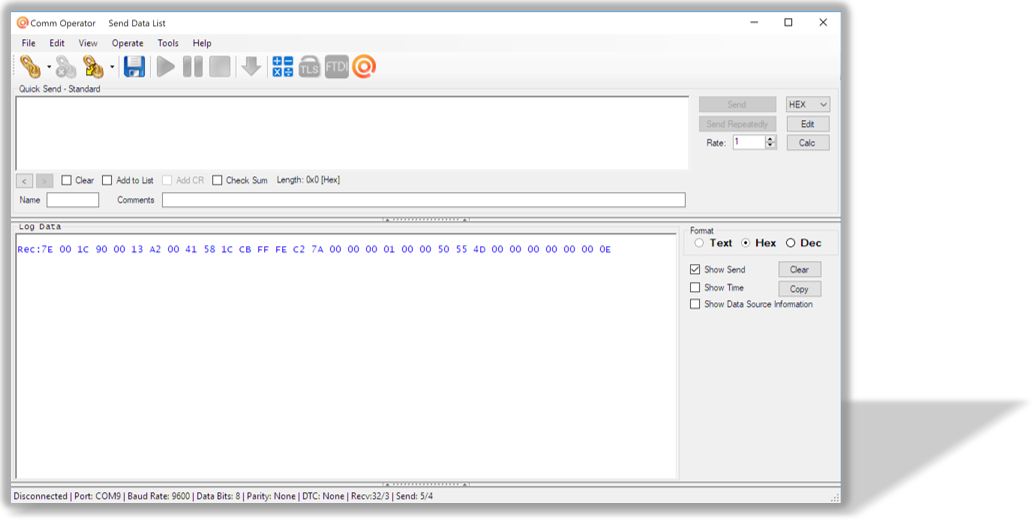
Run Mode
Run mode is the default mode of operation of this sensor. In this mode the sensor sends periodic packets to destination receiver. During the time it is not sending packets, it sleeps and conserves power. Sensor end X-bee operates in API mode and sends packets to the saved destination address on the network specified by the saved PAN ID. Figure 12 illustrates an API packet transmission and reception.
Packet reception at receiver end is ensured by the device by retrying up to 3 times if no acknowledgement is received that the packet has been successfully received. The device uses the acknowledgement functionality available in API mode in X-bee devices therefore user does not need to worry about sending acknowledgements for every packet.
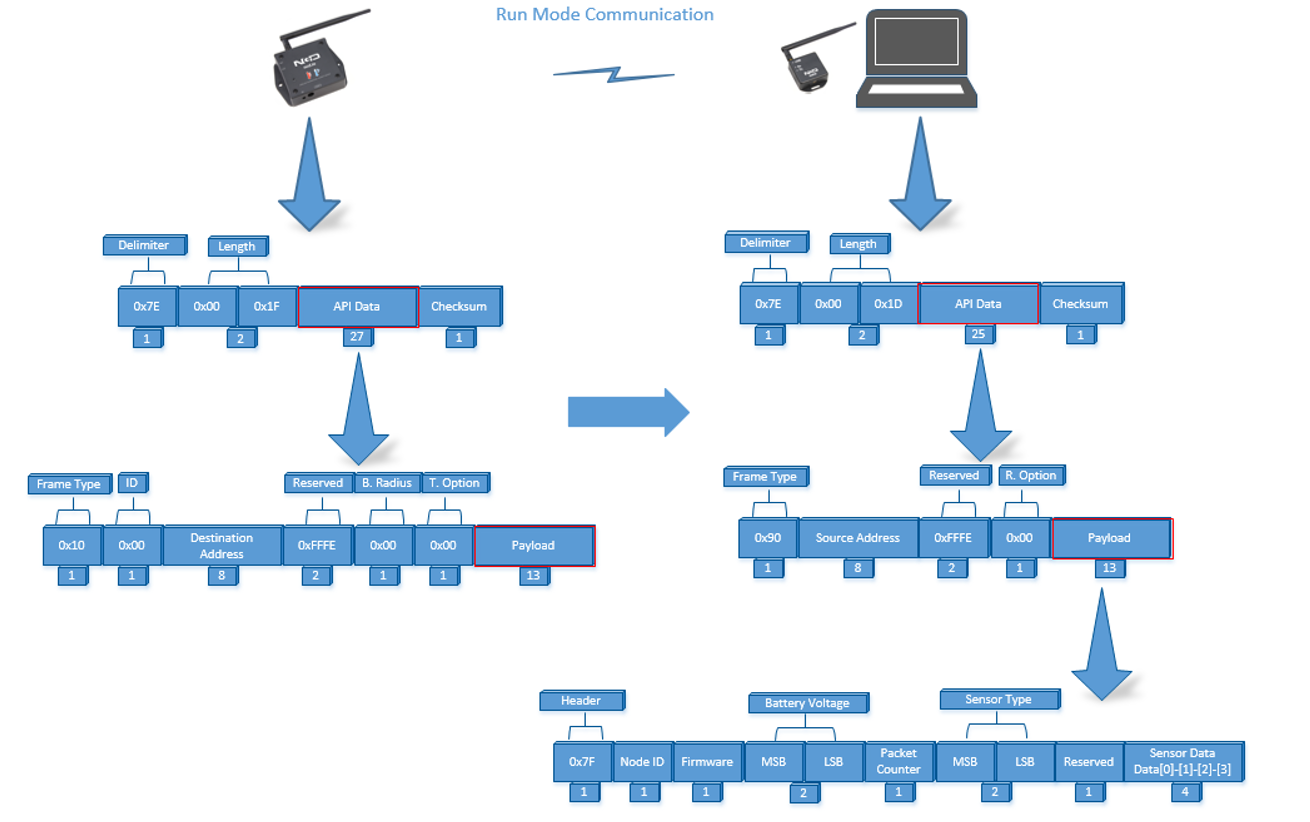
The detail for API packet received at PC end can be read from the X-bee manual available from Digi. The detail of Payload section of packet is shown in Table 3.
Typical response from the device in Run mode is shown in Figure 13 and Figure 14. The utility shown in Figure 14 can be downloaded from the website.
The detail for API packet received at PC end can be read from the X-bee manual available from Digi. The detail of Payload section of packet is shown in Table 3.
Typical response from the device in Run mode is shown in Figure 13 and Figure 14. The utility shown in Figure 14 can be downloaded from the website.
Frame Field | Offset (Payload Section) | Fixed Value (if any) | Description | ||
---|---|---|---|---|---|
Header | 0 | 0x7F |
| ||
Node ID | 1 | 0x00 Factory Default |
| ||
Firmware | 2 | – |
| ||
Battery Voltage | MSB 3 | – | Sampled battery voltage of the device. Battery Voltage=((Battery Voltage MSB x 256+Battery Voltage LSB) x 0.00322 V | ||
LSB 4 | – | ||||
Packet Counter | 5 | – |
| ||
Sensor Type | MSB 6 | 0x00 |
| ||
MSB 7 | 0x20 | ||||
Reserved | 8 | error code |
| ||
Mass Concentration PM1.0 [µg/m³] | MSB 9/ Data[0] | – | Mass Concentration PM1.0 [µg/m³] PM1.0(usigned) = (((Data[0]<<24)+(Data[1]<<16)+(Data[2]<<8)+Data[3])/100.0) | ||
10/ Data[1] | – | ||||
11/ Data[2] | – | ||||
LSB 12/ Data[3] | – | ||||
Mass Concentration PM2.5 [µg/m³] | MSB 13/ Data[4] | – | Mass Concentration PM2.5 [µg/m³] PM2.5(usigned) = (((Data[4]<<24)+(Data[5]<<16)+(Data[6]<<8)+Data[7])/100.0) | ||
14/ Data[5] | – | ||||
15/ Data[6] | – | ||||
LSB 16/ Data[7] | – | ||||
Mass Concentration PM4.0 [µg/m³] | MSB 17/ Data[8] | – | Mass Concentration PM4.0 [µg/m³] PM4.0(usigned) = (((Data[8]<<24)+(Data[9]<<16)+(Data[10]<<8)+Data[11])/100.0) | ||
18/ Data[9] | – | ||||
19/ Data[10] | – | ||||
LSB 20/ Data[11] | – | ||||
Mass Concentration PM10.0 [µg/m³] | MSB 21/ Data[12] | – | Mass Concentration PM10.0 [µg/m³] PM10.0(usigned) = (((Data[12]<<24)+(Data[13]<<16)+(Data[14]<<8)+Data[15])/100.0) | ||
22/ Data[13] | – | ||||
23/ Data[14] | – | ||||
LSB 24/ Data[15] | – | ||||
Number Concentration PM0.5 [#/cm³] | MSB 25/ Data[16] | – | Number Concentration PM0.5 [#/cm³] PM0.5(usigned) = (((Data[16]<<24)+(Data[17]<<16)+(Data[18]<<8)+Data[19])/100.0) | ||
26/ Data[17] | – | ||||
27/ Data[18] | – | ||||
LSB 28/ Data[19] | – | ||||
Number Concentration PM1.0 [#/cm³] | MSB 29/ Data[20] | – | Number Concentration PM1.0 [#/cm³] PM1.0(usigned) = (((Data[20]<<24)+(Data[21]<<16)+(Data[22]<<8)+Data[23])/100.0) | ||
30/ Data[21] | – | ||||
31/ Data[22] | – | ||||
LSB 32/ Data[23] | – | ||||
Number Concentration PM2.5 [#/cm³] | MSB 33/ Data[24] | – | Number Concentration PM2.5 [#/cm³] PM2.5(usigned) = (((Data[24]<<24)+(Data[25]<<16)+(Data[26]<<8)+Data[27])/100.0) | ||
34/ Data[25] | – | ||||
35/ Data[26] | – | ||||
LSB 36/ Data[27] | – | ||||
Number Concentration PM4.0 [#/cm³] | MSB 37/ Data[28] | – | Number Concentration PM4.0 [#/cm³] PM4.0(usigned) = (((Data[28]<<24)+(Data[29]<<16)+(Data[30]<<8)+Data[31])/100.0) | ||
38/ Data[29] | – | ||||
39/ Data[30] | – | ||||
LSB 40/ Data[31] | – | ||||
Number Concentration PM10.0 [#/cm³] | MSB 41/ Data[32] | – | Number Concentration PM10.0 [#/cm³] PM10.0(usigned) = (((Data[32]<<24)+(Data[33]<<16)+(Data[34]<<8)+Data[35])/100.0) | ||
42/ Data[33] | – | ||||
43/ Data[34] | – | ||||
LSB 44/ Data[35] | – | ||||
Typical Particle Size [µm] | MSB 45/ Data[36] | – | Typical Particle Size [µm] (usigned) = (((Data[36]<<24)+(Data[37]<<16)+(Data[38]<<8)+Data[39])/100.0) | ||
46/ Data[37] | – | ||||
47/ Data[38] | – | ||||
LSB 48/ Data[39] | – | ||||
Sensor Data | MSB 49/ Data[40] | – | Humidity data Humidity(usigned) = ((data[0] x 256) + data[1])/100 | ||
50/ Data[41] | – | ||||
51/ Data[42] | – | Temperature data Temperature(signed) = (((data[2] x 256) + data[3])/100)°C | |||
LSB 52/ Data[43] | – |
Sensor Type 53 –
Air Quality CO2 Temperature Humidity Particulate Matter Sensor
The detail for API packet received at PC end can be read from the X-bee manual available from Digi. The detail of Payload section of packet is shown in Table 3.
Typical response from the device in Run mode is shown in Figure 13 and Figure 14. The utility shown in Figure 14 can be downloaded from the website.
Frame Field | Offset (Payload Section) | Fixed Value (if any) | Description | ||
---|---|---|---|---|---|
Header | 0 | 0x7F |
| ||
Node ID | 1 | 0x00 Factory Default |
| ||
Firmware | 2 | – |
| ||
Battery Voltage | MSB 3 | – | Sampled battery voltage of the device. Battery Voltage=((Battery Voltage MSB x 256+Battery Voltage LSB) x 0.00322 V | ||
LSB 4 | – | ||||
Packet Counter | 5 | – |
| ||
Sensor Type | MSB 6 | 0x00 |
| ||
MSB 7 | 0x35 | ||||
Reserved | 8 | error code |
| ||
Mass Concentration PM1.0 [µg/m³] | MSB 9/ Data[0] | – | Mass Concentration PM1.0 [µg/m³] PM1.0(usigned) = (((Data[0]<<24)+(Data[1]<<16)+(Data[2]<<8)+Data[3])/100.0) | ||
10/ Data[1] | – | ||||
11/ Data[2] | – | ||||
LSB 12/ Data[3] | – | ||||
Mass Concentration PM2.5 [µg/m³] | MSB 13/ Data[4] | – | Mass Concentration PM2.5 [µg/m³] PM2.5(usigned) = (((Data[4]<<24)+(Data[5]<<16)+(Data[6]<<8)+Data[7])/100.0) | ||
14/ Data[5] | – | ||||
15/ Data[6] | – | ||||
LSB 16/ Data[7] | – | ||||
Mass Concentration PM4.0 [µg/m³] | MSB 17/ Data[8] | – | Mass Concentration PM4.0 [µg/m³] PM4.0(usigned) = (((Data[8]<<24)+(Data[9]<<16)+(Data[10]<<8)+Data[11])/100.0) | ||
18/ Data[9] | – | ||||
19/ Data[10] | – | ||||
LSB 20/ Data[11] | – | ||||
Mass Concentration PM10.0 [µg/m³] | MSB 21/ Data[12] | – | Mass Concentration PM10.0 [µg/m³] PM10.0(usigned) = (((Data[12]<<24)+(Data[13]<<16)+(Data[14]<<8)+Data[15])/100.0) | ||
22/ Data[13] | – | ||||
23/ Data[14] | – | ||||
LSB 24/ Data[15] | – | ||||
Number Concentration PM0.5 [#/cm³] | MSB 25/ Data[16] | – | Number Concentration PM0.5 [#/cm³] PM0.5(usigned) = (((Data[16]<<24)+(Data[17]<<16)+(Data[18]<<8)+Data[19])/100.0) | ||
26/ Data[17] | – | ||||
27/ Data[18] | – | ||||
LSB 28/ Data[19] | – | ||||
Number Concentration PM1.0 [#/cm³] | MSB 29/ Data[20] | – | Number Concentration PM1.0 [#/cm³] PM1.0(usigned) = (((Data[20]<<24)+(Data[21]<<16)+(Data[22]<<8)+Data[23])/100.0) | ||
30/ Data[21] | – | ||||
31/ Data[22] | – | ||||
LSB 32/ Data[23] | – | ||||
Number Concentration PM2.5 [#/cm³] | MSB 33/ Data[24] | – | Number Concentration PM2.5 [#/cm³] PM2.5(usigned) = (((Data[24]<<24)+(Data[25]<<16)+(Data[26]<<8)+Data[27])/100.0) | ||
34/ Data[25] | – | ||||
35/ Data[26] | – | ||||
LSB 36/ Data[27] | – | ||||
Number Concentration PM4.0 [#/cm³] | MSB 37/ Data[28] | – | Number Concentration PM4.0 [#/cm³] PM4.0(usigned) = (((Data[28]<<24)+(Data[29]<<16)+(Data[30]<<8)+Data[31])/100.0) | ||
38/ Data[29] | – | ||||
39/ Data[30] | – | ||||
LSB 40/ Data[31] | – | ||||
Number Concentration PM10.0 [#/cm³] | MSB 41/ Data[32] | – | Number Concentration PM10.0 [#/cm³] PM10.0(usigned) = (((Data[32]<<24)+(Data[33]<<16)+(Data[34]<<8)+Data[35])/100.0) | ||
42/ Data[33] | – | ||||
43/ Data[34] | – | ||||
LSB 44/ Data[35] | – | ||||
Typical Particle Size [µm] | MSB 45/ Data[36] | – | Typical Particle Size [µm] (usigned) = (((Data[36]<<24)+(Data[37]<<16)+(Data[38]<<8)+Data[39])/100.0) | ||
46/ Data[37] | – | ||||
47/ Data[38] | – | ||||
LSB 48/ Data[39] | – | ||||
Sensor Data | MSB 49/ Data[40] | – | Humidity data Humidity(usigned) = ((data[0] x 256) + data[1])/100
Temperature data Temperature(signed) = (((data[2] x 256) + data[3])/100)°C | ||
50/ Data[41] | – | ||||
51/ Data[42] | – | ||||
LSB 52/ Data[43] | – | ||||
MSB 53/ Data[44] | – | CO2 Concentration in ppm CO2(usigned) = (((Data[44]>>24)+(Data[45]>>16)+(Data[46]>>8)+Data[47])/100.0) | |||
54/ Data[45] | – | ||||
55/ Data[46] | – | ||||
LSB 56/ Data[47 | – |
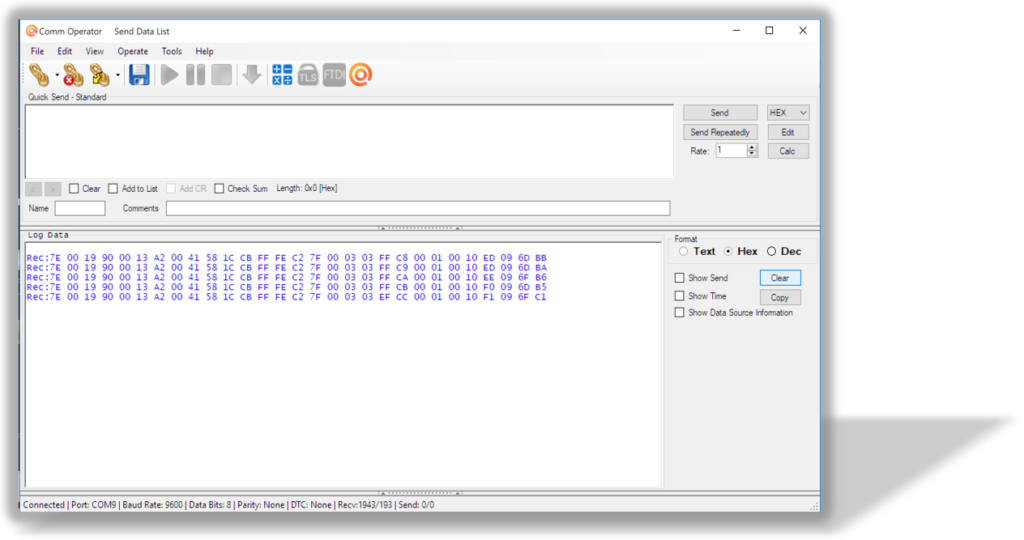
Configuration Mode
Configuration mode is intended to setup the device over the wireless link. Entering configuration mode was already explained in the section “mode selection procedure”. User can also setup X-bee communication and networking parameters using this mode via PC. Note that settings only take effect after reset and are stored inside the device.
In configuration mode, the device sets its X-bee pan id to 7BCD (Hex). Also, the destination address used by the sensor is broadcast (0000FFFF). This ensures that once you put a device in configuration mode you just need to change the PAN ID of your Zigmo to match with sensor and start configuring your device. You can change the PAN ID of your Zigmo using XCTU from Digi. If you use our LabVIEW utility, it will automatically change Zigmo PAN ID once you open the configuration window. When you exit this window your PAN ID will be restored to old value.
A standard configuration packet and its fields are explained in Figure 15. Its possible responses are also shown. The commands supported by this sensor are shown in Table 4, these can be used in the Parameters field of Payload section. The sensor responds to these commands with an acknowledgement if the process completed successfully or with an error if it failed to setup a parameter. The respective Data and Reserve section length and values are shown in Table 5 for the case of acknowledgement. In the case of error, the reserved section will be fixed and not used, while the Error number byte will determine the type of error returned. These errors are mentioned in Table 6.
Figure 15 depicts standard communication between Zigmo/Router and sensor. Sensor commands have variable length frames whereas responses received from sensor are fixed length. The 2 scenarios are also shown, where a command can result in an acknowledgement reception or an error reception at the Zigmo end.
Examples for setting parameters in configuration mode are shown in Appendix A.
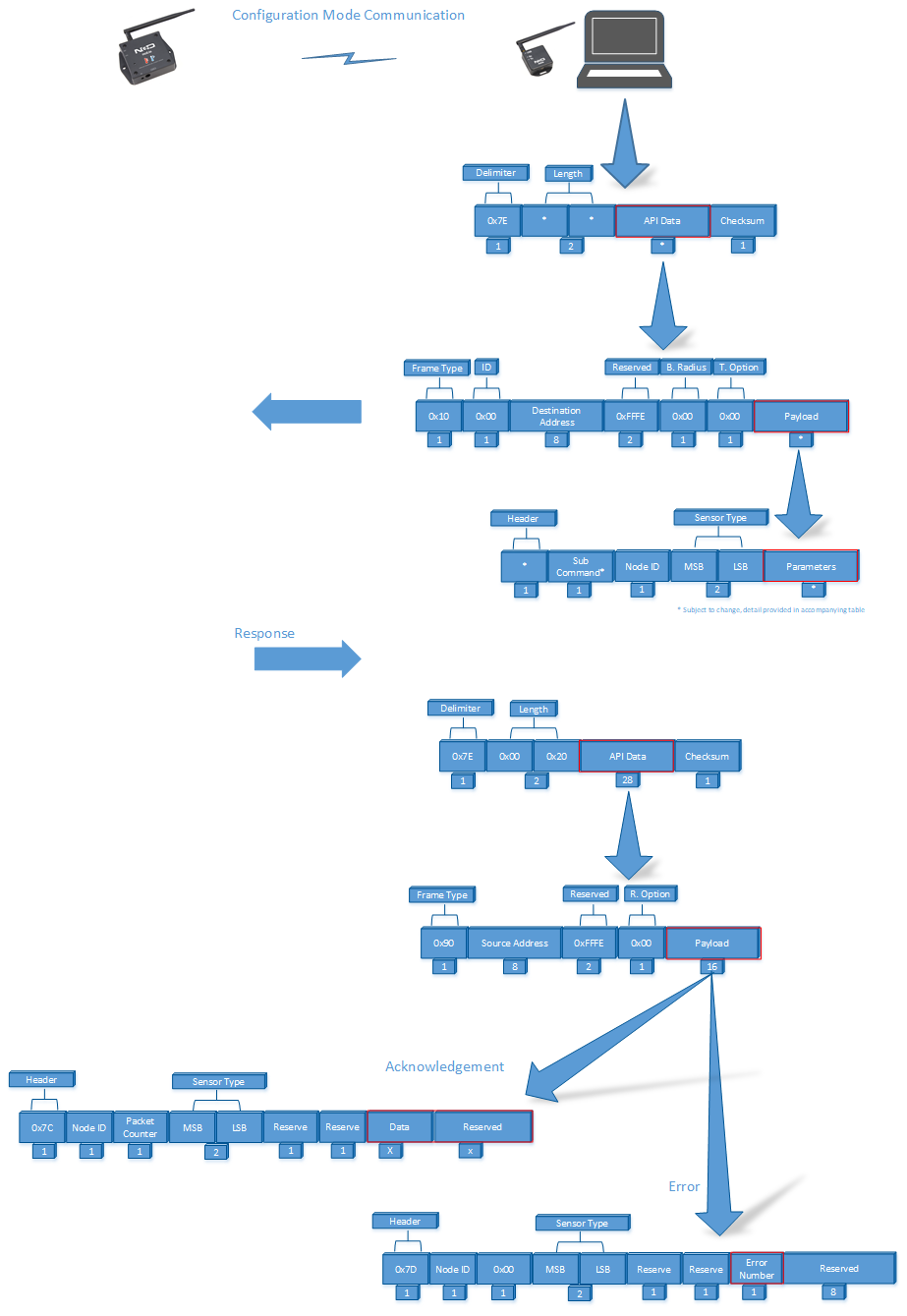
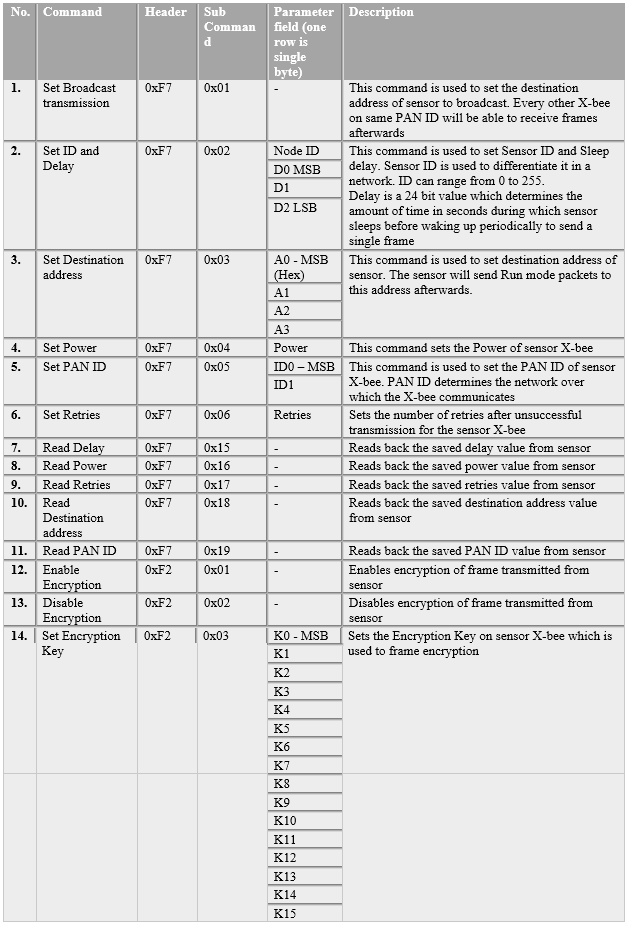
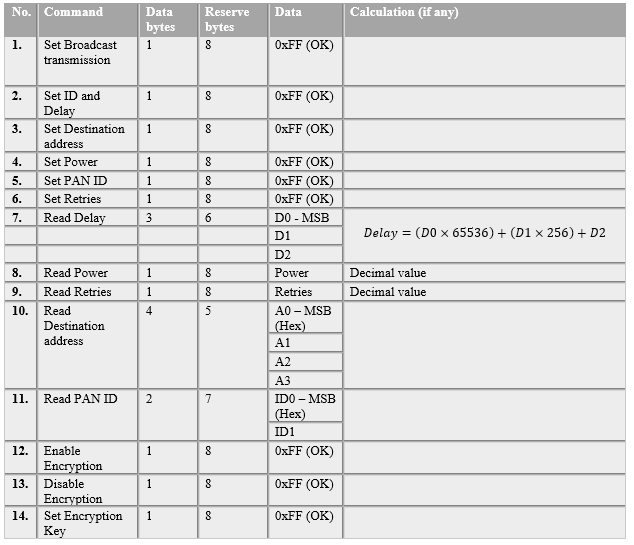
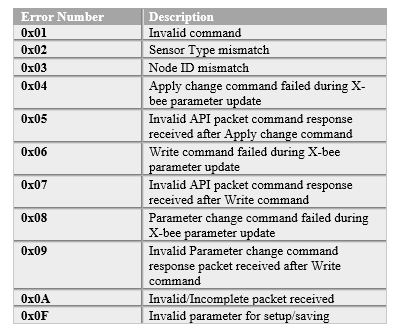
Appendix A
Configuration Commands
1. Set Broadcast Transmission
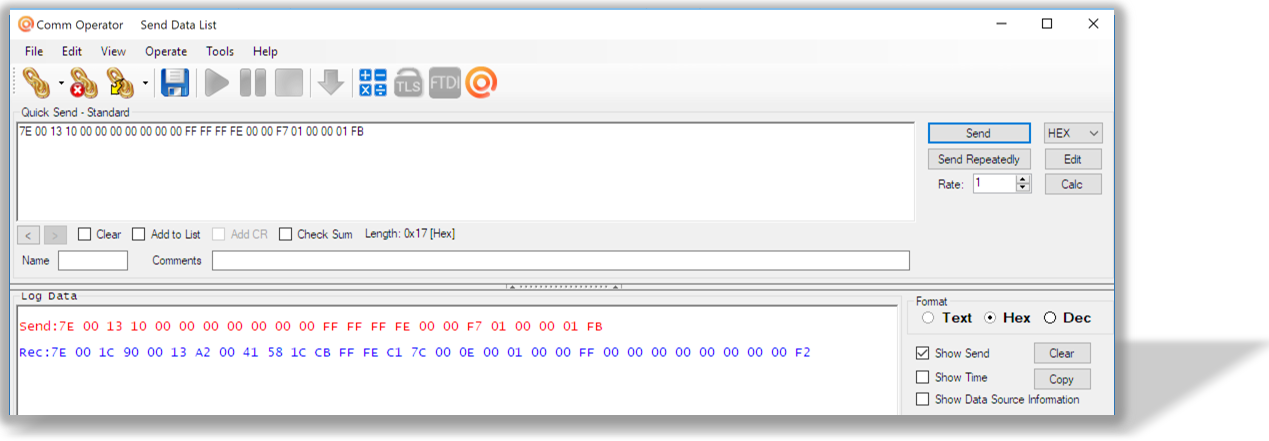
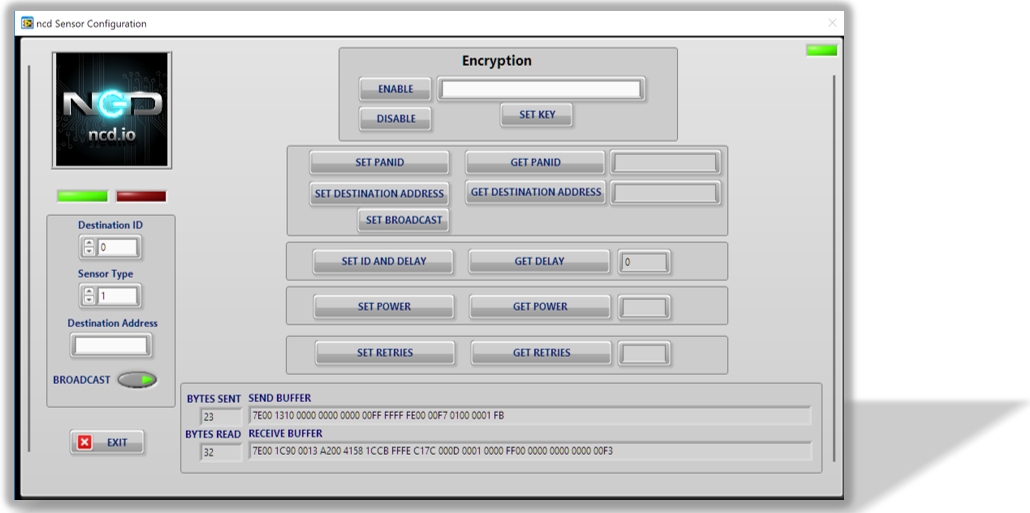
Command For COPY: 7E00 1310 0000 0000 0000 00FF FFFF FE00 00F7 0100 0001 FB
2. Set ID and Delay
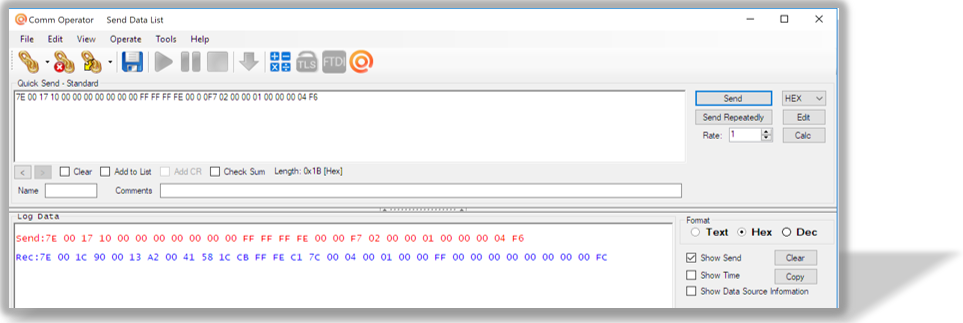
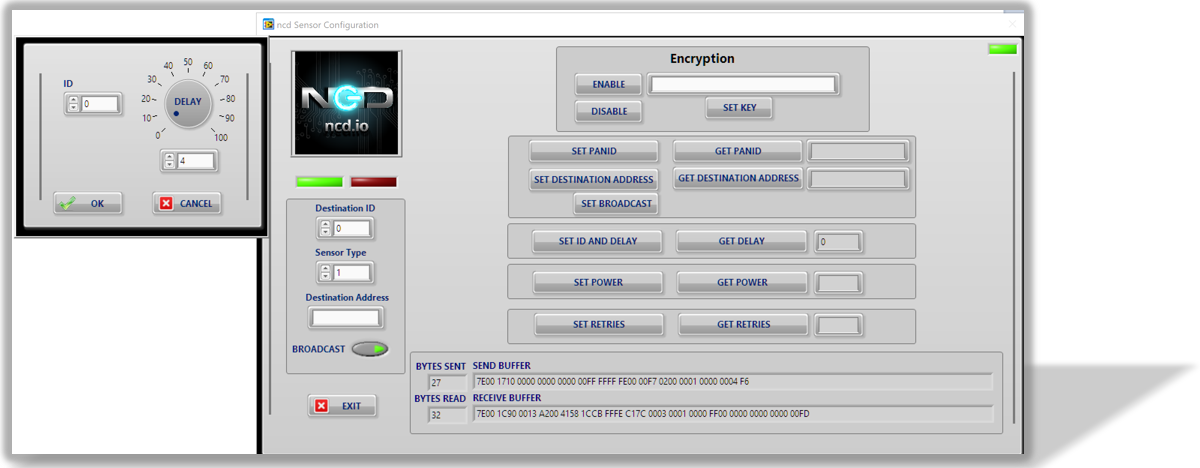
Command For COPY: 7E00 1710 0000 0000 0000 00FF FFFF FE00 00F7 0200 0001 0000 0004 F6
3. Set Destination Address
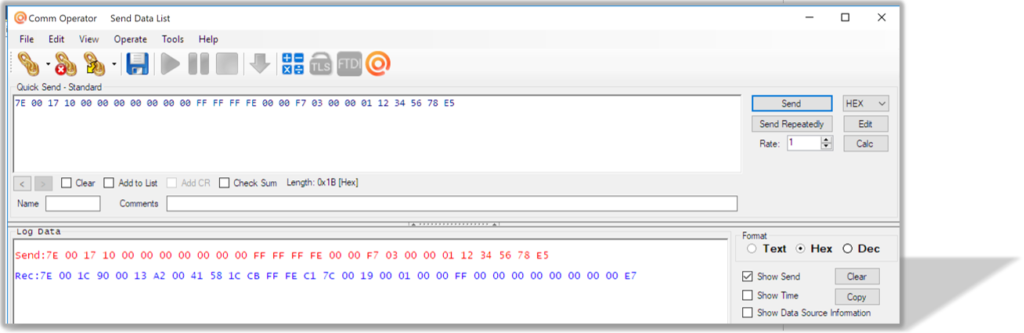
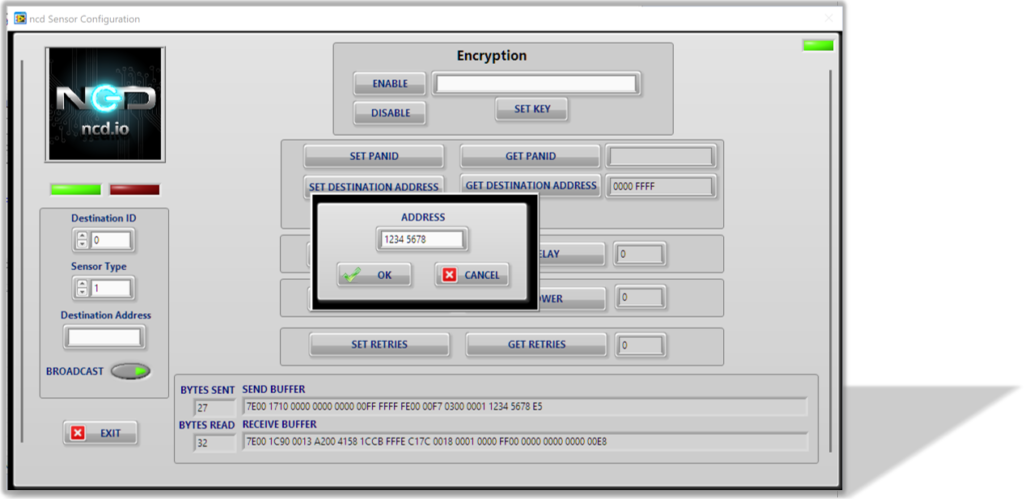
Command For COPY: 7E00 1710 0000 0000 0000 00FF FFFF FE00 00F7 0300 0001 1234 5678 E5
4. Set Power
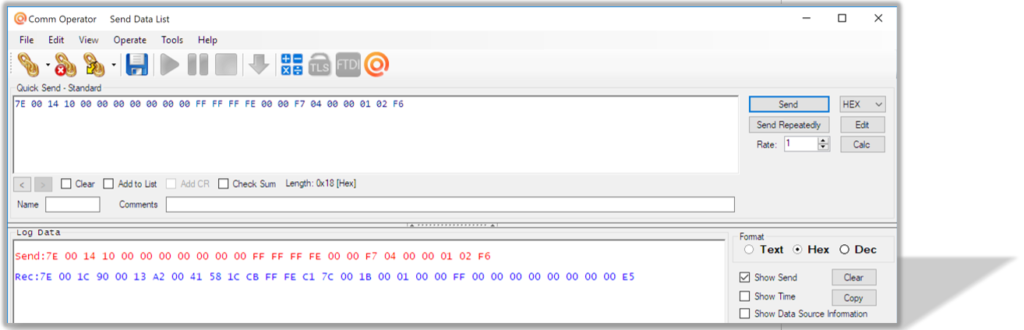
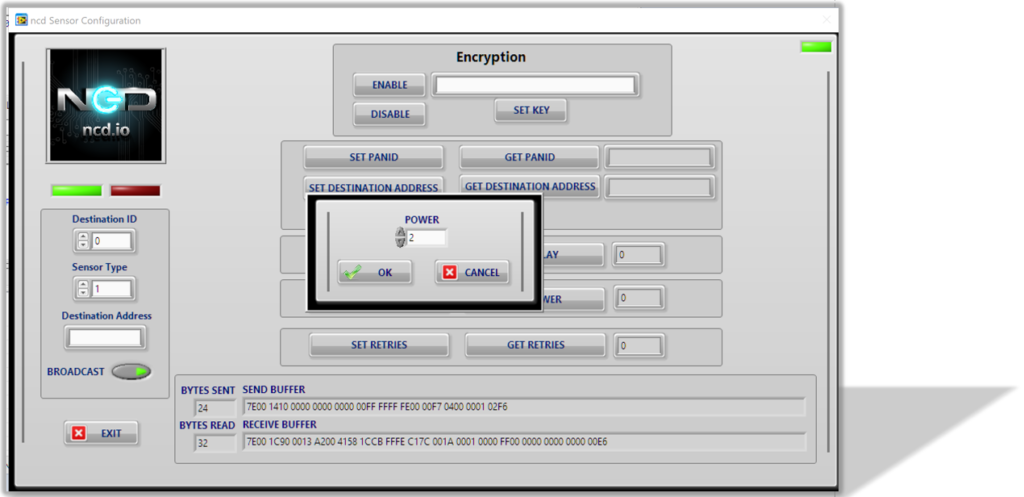
Command For COPY: 7E00 1410 0000 0000 0000 00FF FFFF FE00 00F7 0400 0001 02F6
5. Set PANID
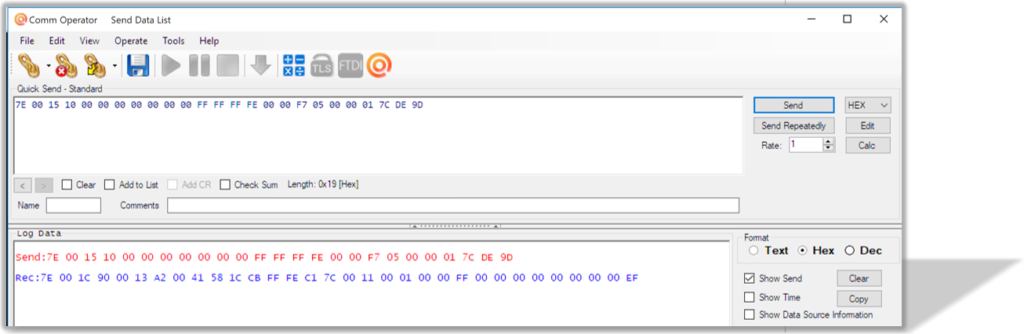
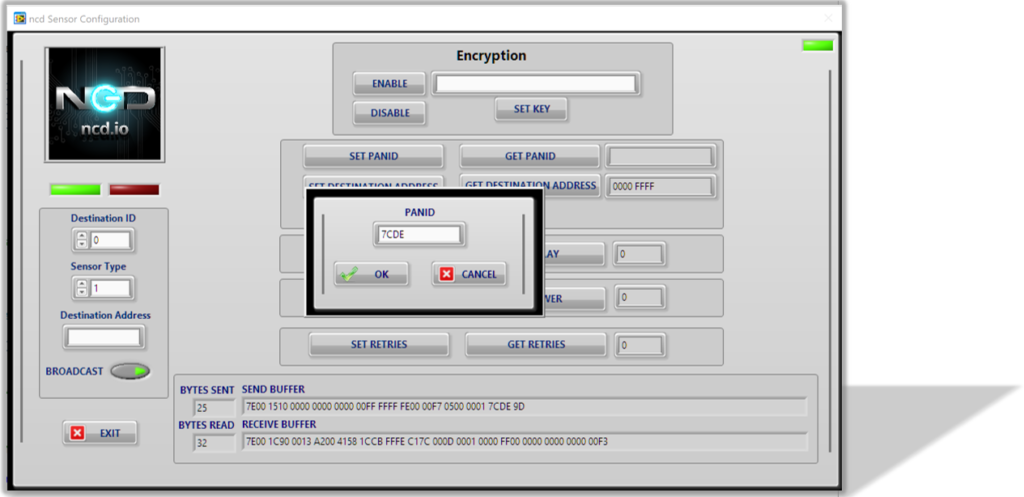
Command For COPY: 7E00 1510 0000 0000 0000 00FF FFFF FE00 00F7 0500 0001 7CDE 9D
6. Set Retries
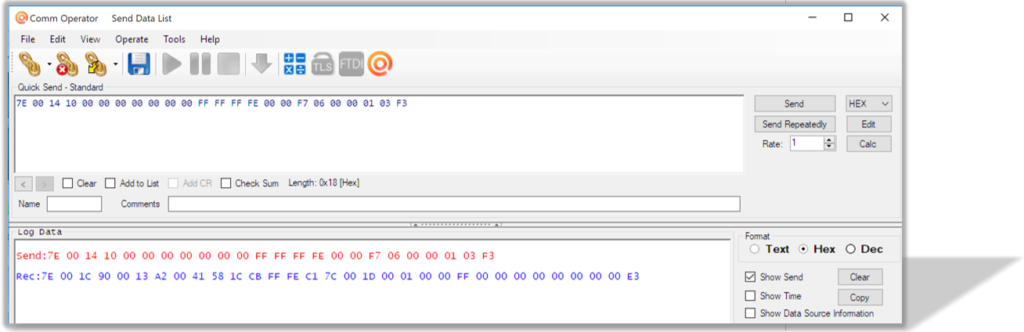
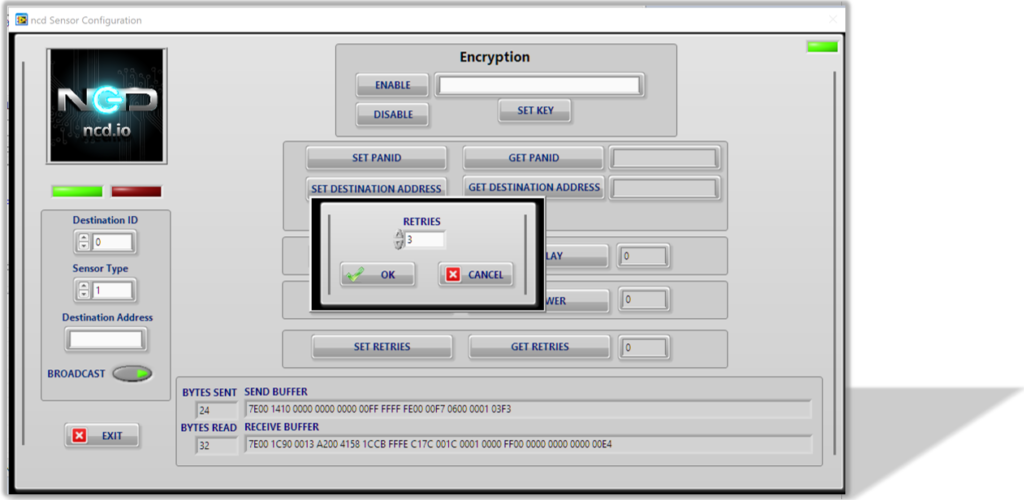
Command For COPY: 7E00 1410 0000 0000 0000 00FF FFFF FE00 00F7 0600 0001 03F3
7. Read Delay
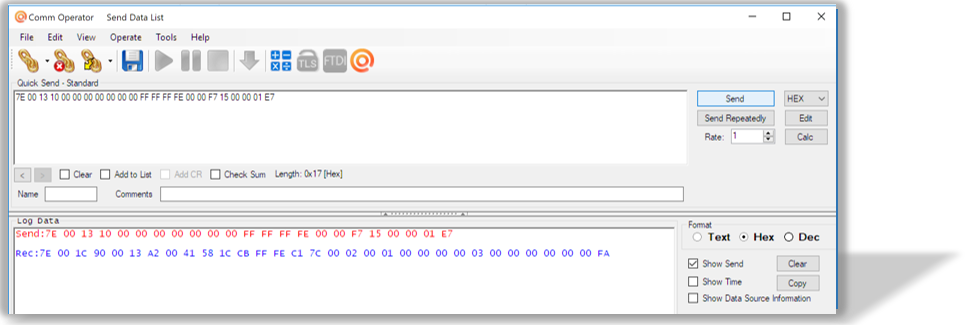
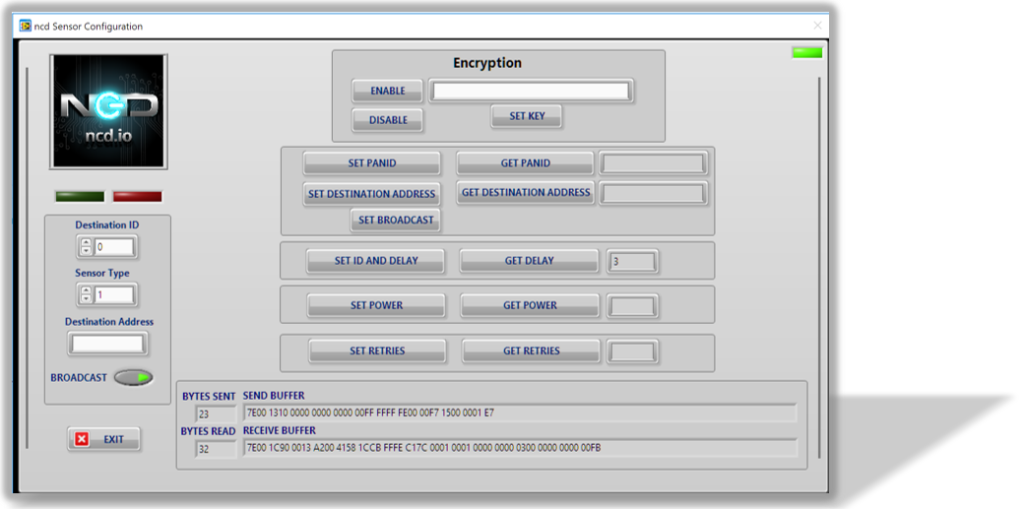
Command For COPY: 7E00 1410 0000 0000 0000 00FF FFFF FE00 00F7 0600 0001 03F3
8. Read Power
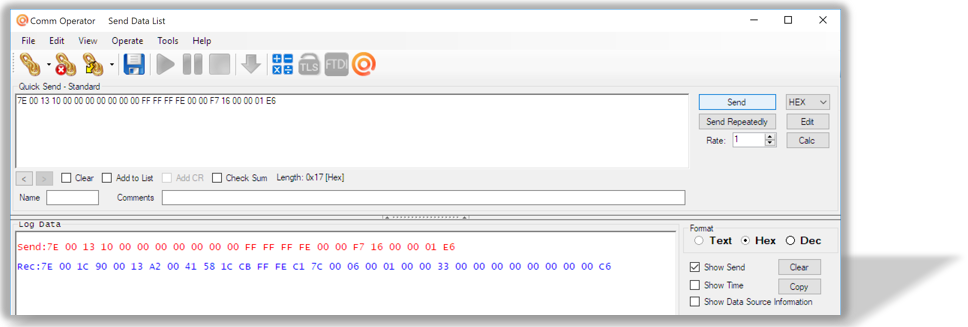
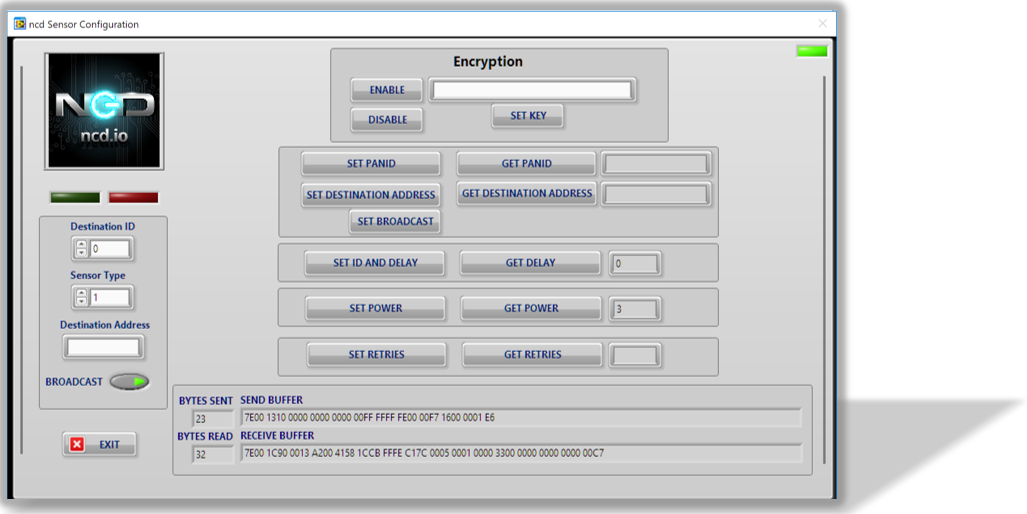
Command For COPY: 7E00 1310 0000 0000 0000 00FF FFFF FE00 00F7 1600 0001 E6
9. Read Retries
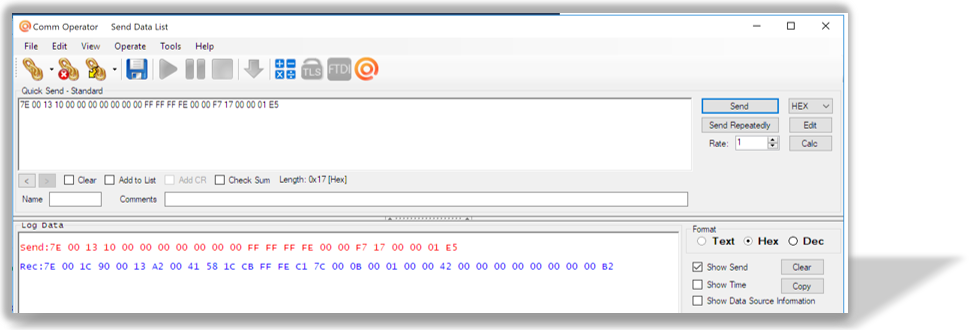
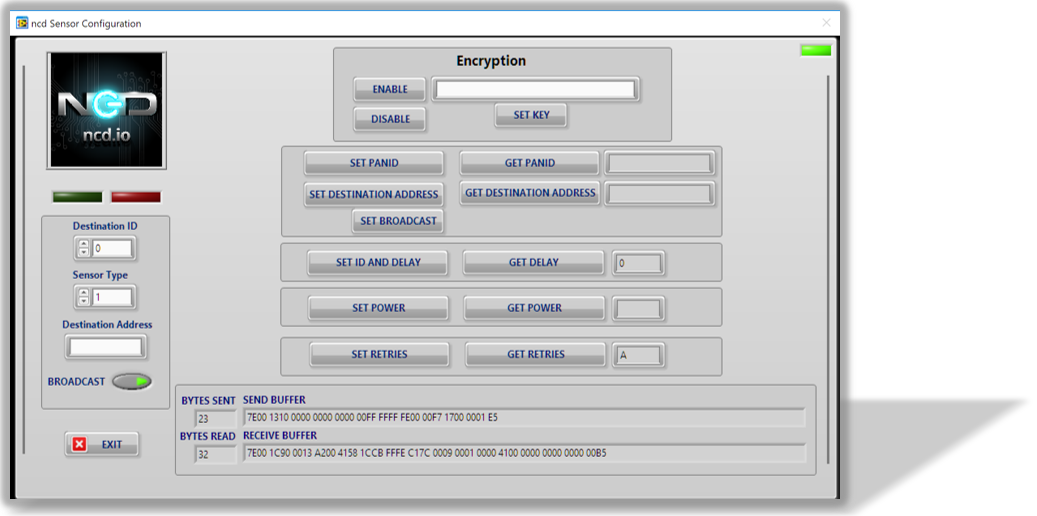
Command For COPY: 7E00 1310 0000 0000 0000 00FF FFFF FE00 00F7 1700 0001 E5
10. Read Destination Address
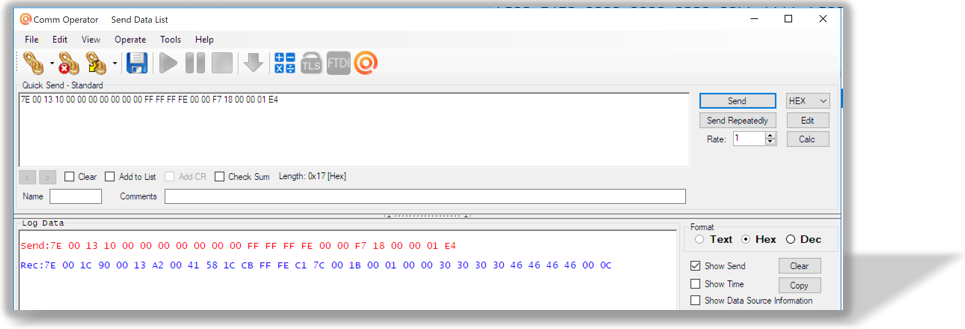
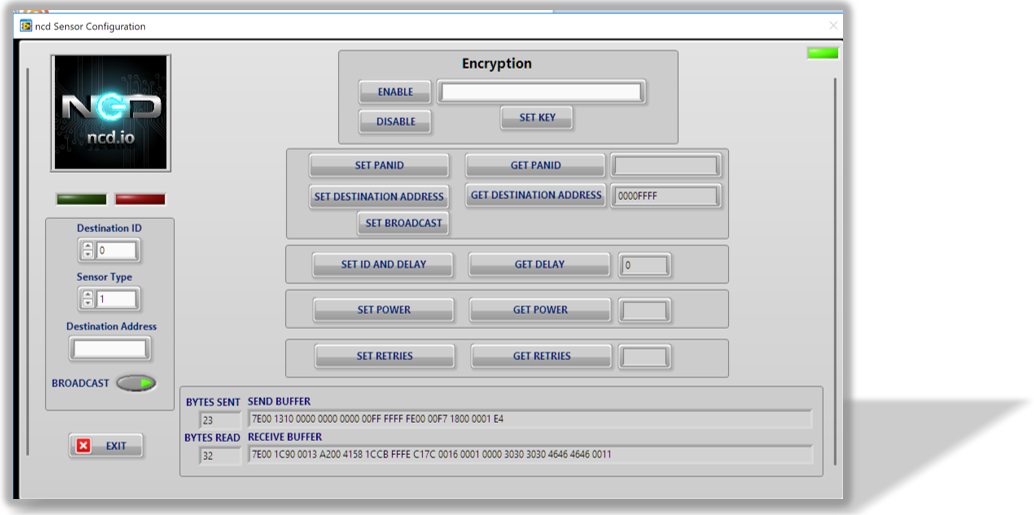
Command For COPY: 7E00 1310 0000 0000 0000 00FF FFFF FE00 00F7 1800 0001 E4
11. Read PANID
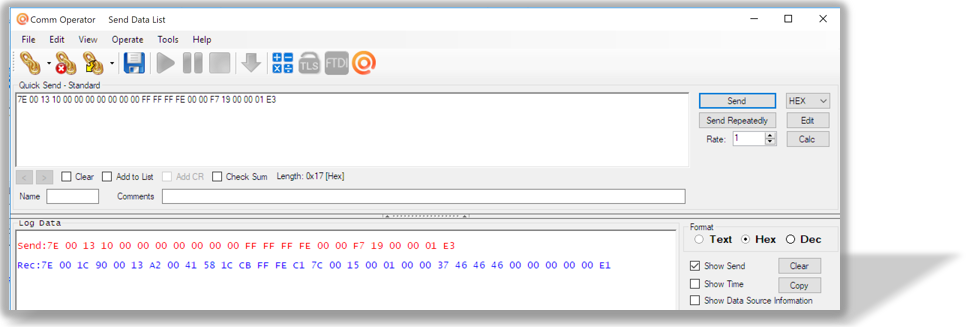
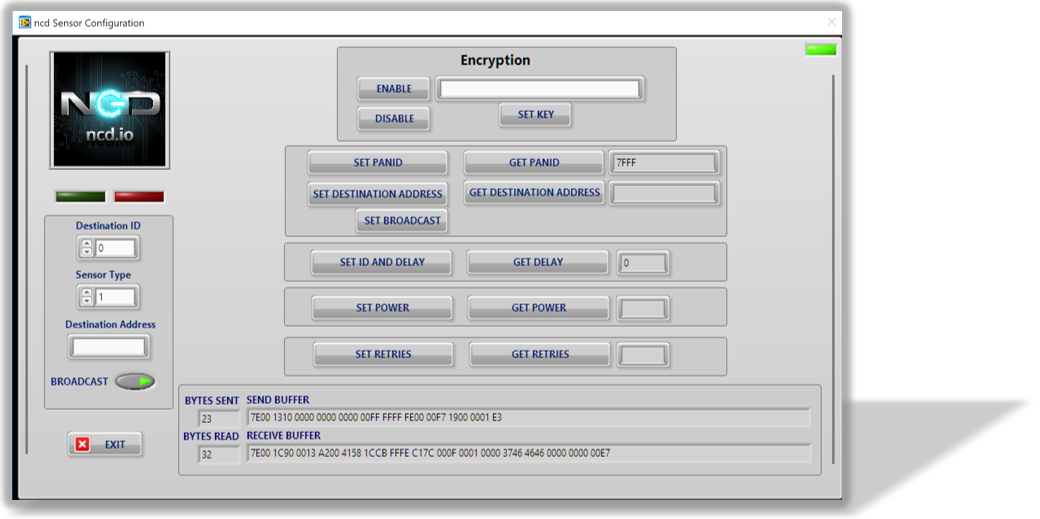
Command For COPY: 7E00 1310 0000 0000 0000 00FF FFFF FE00 00F7 1900 0001 E3
12. Enable Encryption
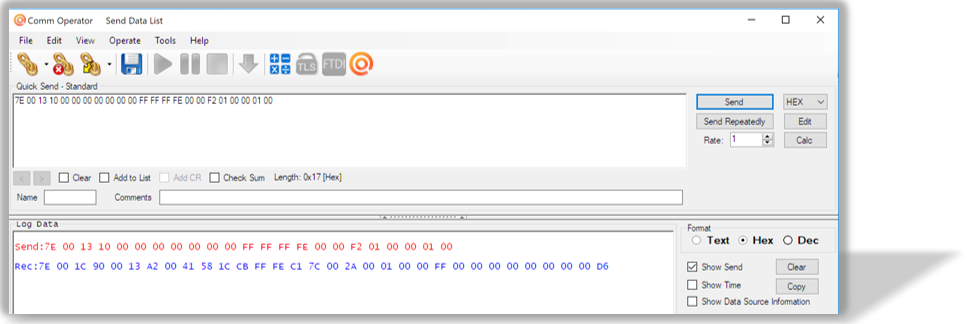
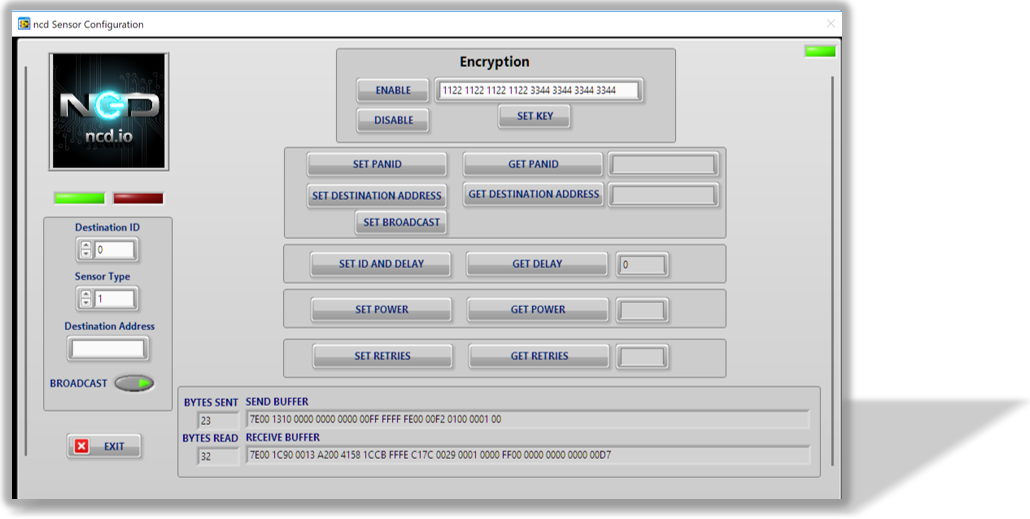
Command For COPY: 7E00 1310 0000 0000 0000 00FF FFFF FE00 00F2 0100 0001 00
13. Disable Encryption
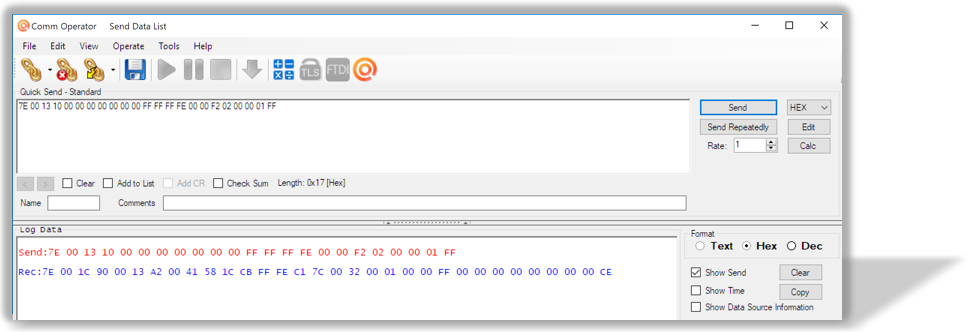
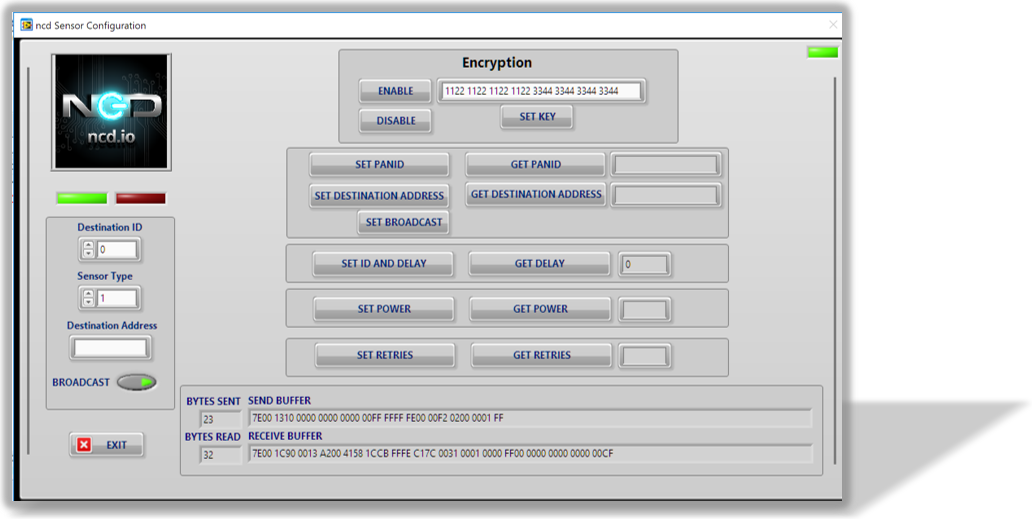
Command For COPY: 7E00 1310 0000 0000 0000 00FF FFFF FE00 00F2 0200 0001 FF
14. Set Encryption Key
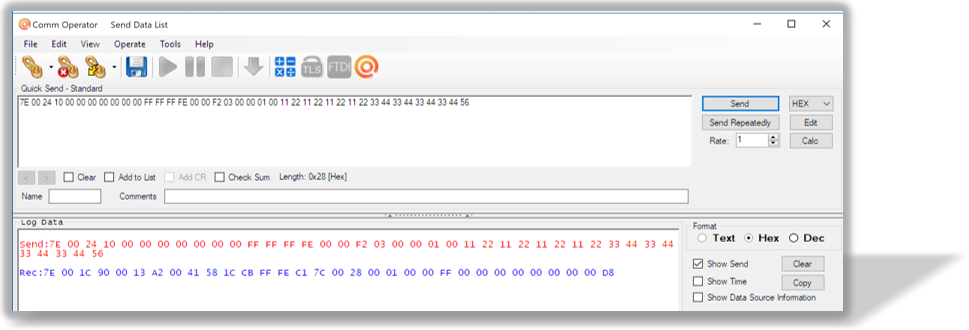
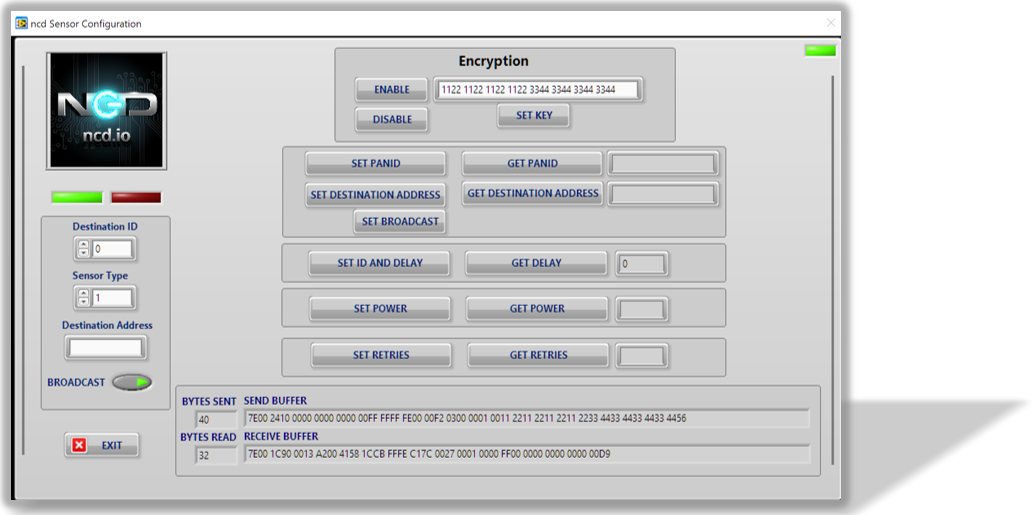
Command For COPY: 7E00 2410 0000 0000 0000 00FF FFFF FE00 00F2 0300 0001 0011 2211 2211 2211 2233 4433 4433 4433 4456
15. Force Sensor Clean
Command For Copy — 7E 00 13 10 00 00 00 00 00 00 00 FF FF FF FE 00 00 F7 1A 00 00 00 E4
Appendix B
Frame Checksum Calculation
In order to successfully communicate over the API protocol, checksum is of vital importance. The X-bee at either end will reject packets if the checksum is not matched. Checksum is also checked by the sensor controller and LabVIEW utility for added security.
For sending packets, checksum calculation works as follows
- Not including the frame delimiter and length, add all the bytes and keep the lower 8 bits of result
- Subtract this value from 0xFF (hex)
- The resultant value is the checksum
- Append this byte to the original packet for sending
Consider the example for the command Set Broadcast shown in Figure 19 in A APPENDIX and see that the calculated checksum matches with the checksum sent by the terminal/LabVIEW

Although checksum is matched by the X-bee itself, but for understanding follow these steps to match checksum at reception
- Not including the frame delimiter and length, add all the bytes including the received checksum
- Keep only the last 8 bits
- If the result is 0xFF, the checksum is correct and the packet can be processed.
Consider the example of the command Set Broadcast shown in Figure 19 in A APPENDIX and see that the received packet checksum verifies since the result is 0xFF.
